Meteorologists and weather enthusiasts are very well aware of the critical role humidity plays in the creation of different meteorological events. But what is humidity, and what are its characteristics?
Humidity primarily refers to the amount of water vapor or water in its gaseous state present in the air at a given time. It is typically represented in relative humidity. Although invisible to the naked eye, high humidity levels can be felt and are often associated with growing levels of discomfort.
At any given time, no matter where we find ourselves (indoors or outdoors), we are constantly surrounded by air containing a certain percentage of humidity.
There is no dispute about the vital role water play in meteorological activities. After all, it is the primary driving force of almost any weather occurrence.
But humidity plays an even more essential role in the transport of water between different regions. This clearly illustrated when one looks at The Water Cycle.
It is also crucial for air to contain just the right amount of moisture to maintain a "balanced" air ratio to benefit the environment and all living organisms. (Too little or too much humidity can negatively impact the environment and even human health.)
In the following sections, we take a closer look at how humidity is caused, how it is measured, and its impact on weather events.
What Causes Humidity?
With humidity clearly defined, one needs to take a close look at how it is caused in the first place and examine its formation:
Humidity is caused when water turns from its liquid or solid state into its gaseous form (water vapor). This occurs due to evaporation and transpiration (known as evapotranspiration), typically resulting from heating due to solar radiation or friction as precipitation travels through air particles.
As radiation from the sun heats the planet's surface, water evaporates from bodies of water (oceans, lakes, dams, puddles) and also from surfaces that are rich in water content like soil. The evaporation process turns water into its gaseous state.
Vegetation in the form of trees and plants also releases water into the atmosphere through their leaves in a process called transpiration. (The combined processes of evaporation and transpiration are called evapotranspiration.)
The evaporation of water is not only the result of solar radiation, though. When precipitation takes place, waterdrops, hail, or snow starts falling towards the ground and accelerate.
As it gains speed, the water starts experiencing drag due to friction with particles and molecules in the air. The friction creates heat, resulting in a significant amount of evaporation to take place, turning some of the precipitation into water vapor,
All these processes are responsible for increasing the humidity in the air.
Facts About Humidity
In earlier sections, the definition and cause of humidity were already established. The following list, though, highlights the key facts and characteristics surrounding this element of weather:
1
Humidity refers to the amount of water vapor present in the atmosphere at any given time.2
Water vapor is nothing more than water in its gaseous state.3
Humidity is caused when water is turned from its liquid or solid into its gaseous state.4
Although humidity and its effects can usually be felt, it is normally invisible to the naked eye.5
Meteorologists use dew points to measure the amount of water vapor present in a body of air.6
Humidity is a crucial element necessary for the formation of snowflakes and hailstones in subzero temperatures, which you can read more about in this article.7
Air contains a certain percentage of humidity at all times.8
Relative humidity is a more accurate reflection of humidity than absolute humidity.
This is a concise and cryptic list of the most important characteristics and facts that define humidity, but the details about specific aspects are highlighted in other sections throughout this report.
Relative Humidity Vs Absolute Humidity
Before explaining how humidity is measured, we need to address the difference between relative humidity and absolute humidity, as there is some confusion about the difference between the two.
Absolute humidity is the measurement of the amount of water vapor in the air. It is a very rigid form as measurement, as it does not take variables like temperature into consideration. It is normally indicated as grams of moisture per cubic meter of air (g/m3).
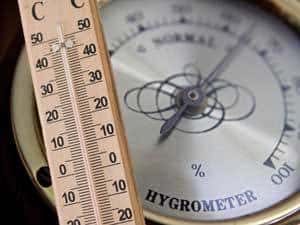
Relative humidity is also the measurement of the amount of water vapor in the air. Unlike absolute humidity though, it is measured relative to the temperature of the air.
In other words, relative humidity measures the percentage of water vapor present in the air relative to the maximum amount of vapor that can be held at a given temperature.
This is important, as warm air can hold much more water vapor than cold air.
If absolute humidity is used to measure the water vapor in both warm and cold air, and it measures identical water vapor percentages in both, it is misleading and not representative of the actual atmospheric conditions.
(In this case, the relative humidity in the cold air is much higher than that of the warm air since warm air holds more moisture than cold air. To get an equal absolute humidity reading, more water vapor must be present in cold air to match the warm air's moisture.)
For this reason, relative humidity is the best and most widely used way of measuring moisture levels as it most accurately reflects the actual atmospheric conditions.
How Humidity Is Measured
The instrument used to measure the humidity in the air is called a hygrometer. There are a variety of these instruments that have been used throughout the years.
The psychrometer is probably the most well-known early example of a humidity measuring device. It basically consists of two thermometers (one being covered with a wet cloth) used to measure the humidity.
The bulb of the thermometer covered by the wet cloth measures a lower temperature as a result of the evaporation of the moisture in the cloth. By using the difference between the two different temperature readings, the humidity is measured.
Obviously, this is not a very accurate and reliable way of measuring humidity.
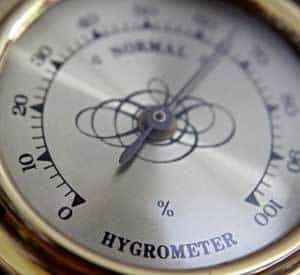
A capacitive or resistance hygrometer uses a much more reliable way to measure humidity. A material able to absorb moisture is used. The amount of moisture influences the material's ability to carry an electrical current.
An electrical current is then sent through the material and measured. Based on the strength of the current (influenced by the amount of moisture absorbed by the material), the amount of humidity in the air can be measured.
The process clearly more complex than just explained. To get a better understanding of hygrometers and how they measure humidity, you can read more about them in this article.
The Effect Of Humidity On The Weather
Humidity is one of the main driving forces of almost all weather systems around the world. Actually, the combination of humidity and temperature is the impetus of many weather systems and occurrences.
The moist air in a warm front gently moves over a cold front and cools down as it rises. It results in condensation and cloud formation, which leads to the gentle precipitation that is welcoming to the agricultural sector. (Similar to the weather produced by a stationary front.)
On the other side of the scale, the warm moist air over the oceans of the tropics rises and forms powerful low-pressure systems. As the air keeps rising and rotating winds are pulled in and building up around the low-pressure center, the warm, humid air keeps feeding the growing system.
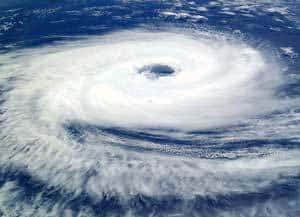
What was a tropical depression can now quickly build into a tropical storm. If enough humid air builds up in this system and stays over the warm ocean waters long enough, hurricanes of varying strength can be very destructive when it makes landfall.
And this whole process got started by some humid air rising up from the ocean's surface. That is why these warm tropical waters are called the engine rooms of big storm systems and the fuel that drives these massive systems.
Similarly, the torrential rains falling during the Monsoon Season over India and Southeast Asia are all part of the circulation pattern that brings huge amounts of humid air from the warm Indian and Western Pacific Oceans during the warm summer season.
As is the case with hurricanes and tropical storms, moisture-filled air is once again one of the main driving forces of a major weather system. This is also another example of how humidity and temperature work together in the creation of a major storm system.
There are obviously many more processes involved in the formation of all these weather systems. If you want to read in detail how hurricanes and monsoons are formed, as well as the role humidity plays in all this, you can read the in-depth article here.
Conclusion
Although it cannot be seen, humidity plays a crucial role in the creation of almost all weather occurrences, as this article clearly illustrated. It highlighted what humidity is, how it forms and also described the different processes involved.
The powerful effect humidity has on the weather on a global scale should also be evident, and we just touched the surface. There is so much more to humidity than covered in this article, but the focus of this post was to capture its definition and illustrate its formation.
Never miss out again when another interesting and helpful article is released and stay updated, while also receiving helpful tips & information by simply clicking on this link .
Until next time, keep your eye on the weather!